2001
Save
or Print a PDF copy of Fact Sheet #08
Average daily stream temperatures are regulated
by many factors: ambient air temperature, relative humidity,
groundwater influx, stream channel morphology (including discharge
rate), and substrate composition (Adams and Sullivan 1989, Brown
1969, Byram and Jemison 1943, NCASI 2000, Patton 1974). Solar
radiation has a relatively small impact on daily mean stream
temperatures (Adams and Sullivan 1989). However, solar radiation
is most responsible for deviations from average daily temperatures
(Adams and Sullivan 1989, Ice 2001), and is almost the only
factor that can be controlled by (active or passive) forest
management. The impact of management change on shade can be
modeled to support the development of better forest management
plans. |
|
Direct solar radiation can be transmitted, absorbed, or reflected.
Only direct solar radiation (not diffused) can possibly affect stream
temperatures (Ice 2001). Riparian vegetation is responsible for moderating
how much direct solar radiation reaches the stream. Complete riparian
canopies transmit about 10 percent of incident short-wave (light)
solar radiation (Black 1985). Increases in direct solar radiation
due to reduction (or absence) of riparian vegetation is most responsible
for high stream temperatures (Barton et al. 1985, Brown and Krygiers
1970, Ice 2001). The only precise method of obtaining solar radiation
input to a stream is to measure it for a specific site at a specific
time (Adams and Sullivan 1989).
Canopy density along the path of incoming direct solar radiation
best describes the ability of riparian forest vegetation to influence
stream temperatures (Brazier and Brown 1973). Canopy density at
any given height in the stand is a function of the stand's composition;
specifically, the crown morphologies of all trees in the stand.
Crown morphology is a function of many variables including: height,
relative dominance, species, and density. Inventory data often contains
measurements necessary for simplified models of crown shapes; while
growth models account for changes over time.
The position of the sun in the sky is a function of latitude, time
of year, and time of day. With this knowledge, the angular altitude
and apparent azimuth of the sun can be calculated. Coupled with
specific knowledge of the riparian system (stream width, stream
slope, stream azimuth, and inventory information), the position
of the sun relative to the stream and canopy can be calculated for
any (and therefore every) time of day.
By varying only the composition of the riparian inventory, comparisons
can be made regarding the impact of management intensity on riparian
shade production. If comparisons between management alternatives
reveal no significant change in shade production, it is reasonable
to conclude that stream temperatures will not be affected. Whether
or not a significant change in shade production will translate into
changes in stream temperature will depend on the aggregate effect
of the variables that regulate stream temperature (listed above)
over the stream reach (which determines the exposure period).
Modeling the effect of management intensity on shade production
requires knowledge of the following dimensions specific to the riparian
system: Latitude, solar declination (time of year), hour angle (time
of day), riparian inventory information (e.g. trees per acre, heights,
crown ratios, crown widths), buffer width, buffer slope, stream
width, stream reach, stream gradient, and stream azimuth. These
variables combine in a unique fashion to create a solar exposure
intensity matrix for every minute of the day, for a given day of
the year.
The following figures illustrate how a model developed for use
with the Landscape Management System accounts for a changes in stand
density (i.e. harvest intensity) on shade production. For this example,
site conditions include: 47º N latitude, +23.5º N declination
(summer solstice), 50 foot wide buffers, 5 percent slope, 15 foot
wide stream, 500 feet of reach, 0 percent gradient, and 270º
azimuth (East/West).
Figures 1 and 2 illustrate the shade produced by symmetric North
and South buffers with identical inventories; the inventory in Figure
1 is relatively dense compared to Figure 2 (see figures for illustrations).
Figures 3 and 4 illustrate the shade produced by North and South
buffers where inventories are asymmetric. Figure 3 has a dense North
buffer and a sparse South buffer; Figure 4 reverses these conditions.
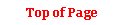

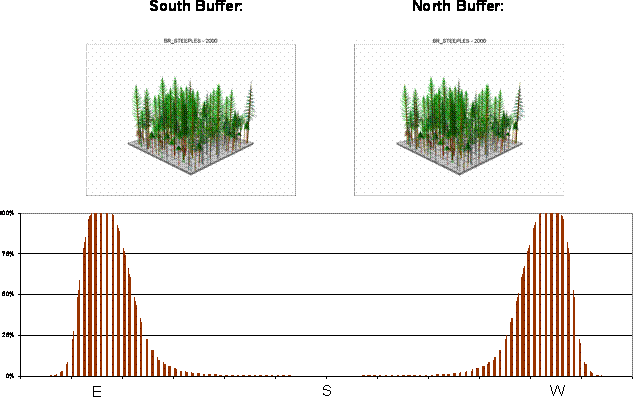
Figure 1. Percent of East-West Stream Reach Receiving Full
Sunlight by Solar Azimuth (Time of Day)
Figure 2. Percent of East-West Stream Reach Receiving Full
Sunlight by Solar Azimuth (Time of Day)
Figure 3. Percent of East-West Stream Reach Receiving Full
Sunlight by Solar Azimuth (Time of Day)
Figure 4. Percent of East-West Stream Reach Receiving Full
Sunlight by Solar Azimuth (Time of Day)
These scenarios illustrate that uniform management prescriptions
will not produce uniform results across riparian landscapes because
of their unique geography. More importantly, uniform management
prescriptions may not achieve the desired results. Grouping riparian
systems by variables that exert the greatest influence on shade
production (e.g. stream azimuth, stream width) can provide predictive
functionality from templates developed using a shade model. Templates
can then be used to design and evaluate management prescriptions
that are appropriately tailored to riparian system-specific conditions
without running models simulations such as above for every possible
scenario.
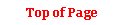

References:
Adams, T.A., and Sullivan, K. 1990. The Physics of forest stream heating:
a simple model. Timber-Fish-Wildlife; Report No. TFW-WQ3-90-007, Washington
Department of Natural Resources, Olympia, Washington.
Barton, D.R., Taylor, W.D., and Biette, R.M. 1985. Dimensions of riparian
buffer strips required to maintain trout habitat in southern Ontario
streams. North American Journal of Fisheries Management. 5:364-378.
Brown, G.W. 1969. Predicting temperatures of small streams. Water
Resources Research. 5(1):68-75.
Brown, G.W., and Krygier, J.T. 1970. Effects of clear cutting on stream
temperature. Water Resources Research. 6:1133-1139.
Byram, G.M., and Jemison, G.M. 1943. Solar Radiation and Forest Fuel
Moisture. Journal of Agricultural Research. 67: 149-176.
Ice, G. 2000. How direct solar radiation and shade influences temperature
in forest streams and relaxation of changes in stream temperature.
Cooperative Monitoring, Evaluation and Research (CMER) Workshop: Heat
transfer processes in forested watersheds and their effects on surface
water temperature. Lacey, Washington, February 2000.
National Council for Air and Stream Improvement, Inc. (NCASI) 2000.
Riparian vegetation effectiveness. Technical Bulletin No. 799. Research
Triangle Park, NC: National Council for Air and Stream Improvement,
Inc.
Patton, D.R. 1973. A literature review of timber harvesting effects
on stream temperature. Research Note RM-249. Rocky Mountain Forest
and Range Experimental Station, USDA Forest Service, Fort Collins,
Colorado.
|